The End of Fossil Fuels: Part 2. Twilight or Dawn?
- Lindsey Grant
- November 23, 2004
- Forum Papers
- Forum Paper
- 0 Comments
Part 1 addressed the period of decline of fossil fuels, lasting perhaps through much of this century,and concluded that the fossil fuel era has been a brief spike that generated an unsustainable growth in population and, in the industrial world, consumption. We are entering an age of overshoot. Humankind may have time for an orderly withdrawal if we have the wisdom to face the change squarely and to unlearn the faith in growth that has grown up in the fossil fuel era. There will be disasters if we do not, and later wisdom may come to see the fossil fuel era as the most intense human disturbance of our natural support systems in history.Part 2 will look toward a much more uncertain future beyond fossil fuels. With the judicious employment of the technologies we have learned – and with a bit of luck – we may be able to create a more harmonious balance with the rest of the biosphere, but only at substantially lower population levels and less consumptive habits. |
Many studies have undertaken to describe how benign renewables might replace fossil fuels. A few of them have noted that population growth makes the task more difficult. Almost none of them turn the issue around and make the point that a smaller population will make it simpler. That is the central point I hope to make.
Once we have left the comforting but dangerous shelter of fossil fuels, we will necessarily turn to biomass, wind, direct solar energy and some more exotic sources. Biomass is traditional, and it is the most versatile form, but it will be a strictly limited resource unless human populations have grown much smaller, and the other renewables in sight can fill only a small part of the energy void, especially if demand does not decline dramatically.
In this speculative world, estimates of one or another source vary by a factor of ten or more. Generally, the high estimates cite a theoretical figure for availability, without considering the costs, the relevance to actual human needs, or the question: can the source yield a net energy gain over the energy put into obtaining it? The low-end figures come from those who study those limits. I am inclined to go with the skeptics. I will cite some numbers that seem reasonable, but will leave it to the footnotes to guide readers to more detailed studies.
Biomass, the Best Hope?
Biomass (particularly fuelwood and charcoal) was and is an important source of energy. At its simplest, biomass is simply fuelwood, which was the major form of energy used in the United States through 1880. Wood and grass are still the principal energy sources in rural areas in the less developed countries. We do not really know how much biomass is used for energy. The World Energy Council (WEC) cites estimates of five to nine percent of total world energy.1 The official figure for the United States is three percent (which is probably low).2
A ton of wood generates about 60 percent as much energy as a ton of coal, and grasses 50 percent. They have been used for fueling steam engines and for industrial power. In Brazil, six million tons of charcoal are used each year in heavy industry such as steel making. Biomass can be liquefied into alcohol and methanol, and it has been used as a motor fuel. Oilseeds and soybeans are easily converted to “biodiesel.” Bagasse, the residue from sugar cane processing, constitutes 3/4 of the weight of the cane. It is used for power and fuel for cane mill operations. In Brazil, India, Mauritius and Thailand, the surplus electricity is sold to the grid.3 As a chemical feedstock, biomass can substitute for fossil fuels. Properly utilized, it generates very little pollution and does not cause climate warming.
Biomass is thus a flexible and benign energy source uniquely capable of filling the roles that fossil energy has filled. But there is a catch: it competes for land and water with food, fiber, and lumber production. There will even be competition from new uses of land to raise chemical feedstocks and pharmaceuticals. And finally, climatologists are proposing the massive expansion of forests as a temporary way of reducing carbon emissions. The worldwide competition for land and water is intense and it is getting worse, because arable land per capita is diminishing everywhere.
A significant early worldwide gain in biomass energy is unlikely, unless the rich outbid the poor and produce commercial energy on land that already is producing food. A long-term gain awaits a dramatic decline in the overall pressure on land and water resources – through a remarkable increase in crop and forest yields or, more realistically, by a turnaround in population growth.
The prospects for biomass energy thus will rise or fall with demographics. With a small enough population, biomass could fill much of the energy gap. The United States is in a relatively good position, for a while. We still have four times as much arable land per capita as the less developed countries, and three times as much as Europe, but our arable land per capita is declining rapidly. In another generation or two, population growth will eat up the grains we now export, unless we find a way to raise yields (which have stagnated) or change our eating habits, and after that comes the question, how do we feed a growing population? The competition for land is coming home to the United States, and that does not look promising for biomass energy.
There are some practical opportunities in the United States. The commercial pine plantations in the Southeastern United States produce lumber, paper and pulp. They can be harvested for energy if energy prices rise enough to compete with them. But there is a long term threat: models of global warming agree that the Southeastern U.S. will be getting hotter and drier, and probably turning from piney woods into savannah – and that is not a good portent for the pine plantations. This is an argument not usually heard for stopping anthropogenic climate warming. In the American West, we have learned from recent disastrous forest fires that softwood stands have become much too dense, as a result of bad management for two centuries. As an interim measure, those stands should be thinned and the biomass harvested.
Some biomass energy could be gained from unused crop residues, forestry residues (scrap lumber and sawdust) and urban wastes that are presently wasted because we do not have the management systems and market demand to put them to use. In the European Union, “pollution taxes” are being used to penalize fossil fuels. We could use the same approach to promote the more systematic use of renewables such as biomass. That could provide the foundation of a strategy for the transition into new energy sources.
There are limits. First, biomass is a diffuse energy source. Until petroleum began to run down, we had simply to drill a hole and let a highly concentrated form of energy flow out. No more. Biomass usually converts about 0.1 percent of the solar energy striking plants into usable energy through photosynthesis. Unless the biomass is a byproduct, like bagasse, it is expensive to harvest. It must usually be fertilized to justify the cost of harvesting. And some of the biomass will need to be converted into motor fuels to harvest and transport the biomass itself, or recycled into fertilizer to raise more biomass (because commercial fertilizer is now made from fossil fuels which will not be available after the energy transition). Less energy-dense stands do not pay back the energy used to raise, harvest and process them, unless they are harvested, traditionally, by peasants who have no opportunity costs for their labor. (The U.S. Government subsidy of ethanol alcohol from corn — the grain, not the stalks — was dictated by politics rather than economics. Most experts believe that it produces less energy than went into making it.4)
Second, biomass is not necessarily benign. Burning it in traditional faulty stoves or fireplaces can make it a killer, or at least a potent health problem. (I remember visiting Himalayan peasant huts and being driven out by the wood smoke.) We hardly want to go back to the good old days. And, unlike oil or natural gas, biomass harvesting can strip the tree cover and denude the land, as growing populations attempt to supply their energy needs. That is a pretty fair description of the poorer countries now. Fuelwood and grass are collected from marginal woodlands, hillsides, roadside and village trees, orchards, rubber plantations and grasslands. It is hard work, often done by young women close to the economic margin. We don’t want to perpetuate that scenario. It is hardly the prescription of the future that most of us have entertained, but it may necessarily still have a role because it is better than no energy. That again is a function of crowding. With a better population/resource ratio, there will be sufficient land and water to get the required biomass from less brutal collecting practices.
Third, the annual flow of sunlight imposes limits to biomass energy production. Fossil fuels are the biomass of earlier eons, and we have been burning them to support our energy habit – perhaps twice as fast as the Earth’s total annual solar input via photosynthesis. In the future, that annual budget must support all the world’s life, including our own needs for food, fiber and biomass energy. Humans already use a substantial portion of total photosynthetic energy5, taking it from the other living creatures. We cannot use more biomass energy without imperiling the biosphere and ourselves, unless we sharply reduce our other demands on land and water resources. And mathematically, the upper limit will be far below present brief spurt of fossil energy production.
How far below? From the welter of estimates of biomass potential, let me analyze one that is near the middle of the pack – that 350 million hectares could produce 80 Exajoules (EJ) of energy per year, about one-fourth of current total world energy consumption.6 Corn may yield about 15 tons of biomass per hectare, which is consistent with that estimate. However, most farmland produces much less biomass. Since 350 million hectares is one-fourth of total world cropland; a more realistic figure for average biomass production would be eight tons or less, thus halving the gross energy estimate to 40 EJ.
Even that estimate is unrealistic under present conditions, because the world cannot afford to turn over a quarter of its diminishing cropland to biomass energy production; it needs that land for food and fiber production. We could get part or all of those 350 million hectares from tree plantations on presently underutilized forest land, but that would cut the energy production to much less than 40 EJ, because trees grow more slowly than grasses, and their biomass yield is unlikely to exceed three tons/hectare – especially since the land available will be marginal. Such land is unlikely to add much to the present biomass harvest – part of which already comes from peasants cutting wood on that land. It would take heavy fertilization and management to raise the production, and even then the harvest might not justify the energy inputs.
For the United States, I have pointed out that our situation is relatively good, but declining fast. Toward the end of the century, when we will need the biomass, anticipated population growth will have ended our food surplus and wiped out any prospects for biomass energy.
Demographics is the key, in the United States and elsewhere. A world of half the present 6.4 billion could release a quarter of arable land to biomass energy production, and still have 50 percent more arable land per capita for food production. That may not be enough, in a world without fossil fuels for fertilizer production (see Agriculture below). If we halve the population again, to 1.6 billion (back to the 1900 level), there is ample land for food and energy production. We would be better off than in 1900, because we have learned better ways of using land and water.
The world got by on biomass for almost the entire span of human existence.
The Speculative Sources
One can understand the near-desperation with which people look toward wind, solar and hydrogen, given the limits on traditional fuels, but wishing is not necessarily enough.
Wind. To a point, wind is the next best hope. The technology is in place, and its cost (at the wind turbine) is in the neighborhood of 5¢/kWh (kilowatt hour), which is about one-third higher than conventional power in the United States. One regularly hears statements that there is enough wind in the United States to provide some multiple of our total energy needs, but they apparently are based on a rough theoretical calculation of all the wind energy in the United States, ignoring the question: what kind of wind?
The energy in wind varies with the cube of the wind speed. An 8 meter per second (mps; i.e.18 mph) wind has half again as much energy as a 7 mps wind. Commercial wind energy becomes practicable only at about 7 to 8 mps. Only in limited locations does the wind blow regularly enough and hard enough to make wind turbines produce more energy than it takes to build and operate them. The American Wind Energy Association (AWEA) has made grandiose claims, but its practical target is much more modest: to supply wind power for six percent of U.S. electricity in 2020, i.e. 2.4 percent of anticipated total energy needs.
And most grand claims for wind power ignore the question: What do you do when there is no wind? I will discuss the problem of intermittency later. One cannot predict very accurately when the wind will blow. Even if one could, it is unlikely to blow in synchrony with power needs. It becomes very difficult to incorporate erratic wind power into a conventional grid once the proportion of wind power passes 20 percent. Germany is approaching that level, now, and Denmark has stopped adding wind power.
While fossil fuel energy is available, expansion beyond that line may not be economical. When fossil fuel is gone, wind may be the best of some bad choices. Utilities regularly maintain excess gas-fired capacity, which stands idle most of the time, to meet peak load demand. Wind may eventually help to meet that demand, or perhaps to power some form of energy storage. However, the shortage of good sites will probably limit our wind energy output.7
A shift to wind will be expensive. Wind turbines generate only about 20-30 percent of their rated capacity, because of erratic winds, while a coal-fired plant may operate at 90% of capacity. The installed costs of both types of plant are roughly similar, so the capital costs of wind energy will be three or four times that of the coal plant.
Wind power is relatively benign, environmentally. One problem is the noise, which can be heard for over a mile from the new large turbines. Europeans, accustomed to their tidy landscapes and population densities, find that a serious problem. In the United States, the best wind sites are offshore, in certain low mountain passes (“low” because air density decreases with altitude), and in the High Plains. In most such locations there are not too many people to object to the aesthetics – if indeed a row of windmills on a lonely ridge in West Texas is thought to disfigure the landscape. Another problem is the slaughter of birds. With the modern enthusiasm for electronics, tens of thousands of towers are going up all over the United States (and we assume more elsewhere). The Audubon Society complains that millions of birds are being killed annually, and the addition of thousands of huge wind turbines to the landscape would multiply the problem. One can only hope, rather helplessly, that some way will be found to shoo the birds away from such structures. How long does natural selection take to develop birds that will dislike the towers?
Nevertheless, as conventional energy prices rise, wind seems a good bet – for some of our power.
Direct Solar. Solar energy, because it is the object of so much enthusiasm, is the subject of wildly varying expectations. I will thread my way through some of the more cautious speculation.
Photovoltaic energy is growing, but from a tiny base of less than 0.0006 of U.S. energy production. It is already useful as a niche source of energy, primarily for stand-alone applications needing very little electric power. It has been touted as the “energy of the future”, but it may never replace most of the power that fossil fuels routinely put into the grid. Right now, subsidized by favorable state legislation, solar enthusiasts are reselling power to the grid at about $0.10 per kWh, three times the utilities’ wholesale price, and still the enthusiasts are losing money. A student of renewables, Ted Trainer, has estimated the cost of building and operating a solar plant in Australia over 30 and 25 year life cycles and found it 33-47 times as expensive as a coal plant, even before factoring in the high maintenance costs of solar power.8 Another student, Andrew Ferguson, points out that even if enough direct solar capacity could be built to meet peak U.S. electricity demand (because peak demand and peak sunlight coincide), a perpetual annual investment of $110 billion each year would still provide only 24 percent of present U.S. total electricity needs, because solar power functions intermittently.9
I would add that 24 percent of electrical demand is just 10 percent of total U.S. energy demand, and that his $5/watt capital cost estimate is five times that of wind power. And Ferguson is describing only the capital costs, not maintenance and cleaning (dust on the panels kills their efficiency; and solar works best in sunny, and therefore dry and dusty, locations). He did not include the cost of the capital, distribution costs and the energy losses associated with moving it to distant locations on the grid, or converting it to AC.
Advocates promise that costs will come down. Skeptics point out that most of the cost of a solar plant is the supporting structure, that there are few technological gains in sight there, and that the price will rise as energy costs drive up the price of the materials.
Photovoltaic power demands a lot of space. To stake out a maximum: the area of solar collectors needed to provide all present U.S. power needs (intermittently) would be 15,000 square kilometers10 (5800 square miles). That figure should probably be quadrupled in order to spread out the panels to prevent them from shading each other, and to provide space for support structures and access lanes. That would monopolize an area almost one-fourth the size of New Mexico. The birds, lizards and environmentalists will not be happy.
Photovoltaics may be more successful as part of a mixed energy future, generating “distributed power” such as electricity for household use in sunny climates, storing the energy in batteries for night time or cloudy weather. It will be very expensive electricity, and it won’t run many air conditioners or heaters. Perhaps more important over the long term is passive solar energy — the use of sunlight to warm buildings by properly designing and placing them. From personal experience, I know the potential savings. This is a marginal role, however. Home heating is a tiny fraction of U.S. energy consumption, most housing is badly designed for passive solar, and it will take generations to replace the present housing stock with solar houses.
Solar thermal power, another form of direct solar, is designed for grid use. It uses sunlight to heat an oil or salt energy carrier, which is then used to generate steam with a conventional boiler. One such plant in California (with some conventional backup) produced power for the grid at a cost of 8-10 cents/kWh, over twice the price of conventional power, but went bankrupt. At one time, it was said to be the producer of 90 percent of the direct solar energy actually delivered to the grid, worldwide. Such a plant would need about 92 square kilometers (33 square miles) to match the output of a conventional 1000 MW fossil fuel plant.11 A coal plant takes only a few acres, but the difference narrows when one includes the coal mine and the dedicated railway line that brings coal to the plant, and solar thermal is much less disruptive to the environment. Despite its misadventures, the California solar thermal plant may show the way to a useful renewable electricity source. Expensive, but all power will be expensive.
Intermittency and the Storage Problem: the Hydrogen Dream. The bugbear of wind and solar energy is their erratic nature. Modern societies want reliable energy. Renewable energy enthusiasts propose that excess wind and solar generating capacity be used to store the energy for later use. The question is, how?
There have been various ingenious proposals: storing heat in salt; compressed air stored in abandoned mines; giant flywheels; and pumped storage, which is simply water pumped back up into a reservoir with excess energy when demand is slack, to be run through the hydroelectric turbines again. (The loss of energy in that transaction is justified by the price differential between peaking power and slack demand periods.) Pumped storage is practical and already in use in limited situations; the other proposals are speculative and probably inordinately expensive.
The “hydrogen economy” dream is a mixture of two different goals: to use hydrogen to store renewable energy; and more immediately to find a replacement for gasoline. The idea is to extract hydrogen from water and use it as a fuel. Fed into fuel cells (when they become practical), it would provide clean power more efficiently than the internal combustion engine. And, in this dream, the electricity could be fed into the power grid, thus solving the problem of intermittency.
That seems reasonable, at first. Hydrogen is the most common element in the known universe. The Secretary of Energy once exulted that “hydrogen is free!” But that is far from true. We are learning some of the problems. Water (H2O) is a tenacious molecule, and nobody has yet found a way to split it and sequester the hydrogen except with energy inputs far exceeding the potential output. That means that hydrogen is not a source of energy unless someday we should learn to extract it from water with a net energy gain. Failing that, it is simply an energy carrier – a way to store energy until it is needed. And it is an intractable element. It is the lightest of gases, and a huge volume holds little energy compared to conventional energy sources. To use it, particularly for transportation, it must be liquefied by cooling it to nearly absolute zero, and keeping it there – which may take 30 percent of the energy in the hydrogen – or stored under pressures up to 10,000 psi (pounds per square inch), which also requires a lot of energy and demands an enormously strong tank much larger than present gasoline tanks, which converts every vehicle into a mobile bomb. Hydrogen is hard to transport and prone to leakage, which of course dissipates the energy gained and which may eventually become a problem in the atmosphere. It would be hard to transport, since it turns metals brittle. It ignites at a dangerously low temperature.
We can indeed isolate hydrogen. It is already produced for specialized industrial uses. But it is expensive. It wholesales in my state for $98 per 1000 cubic feet, in cylinders. By contrast, the utility company delivers that much natural gas for $6. The price ratio is 16:1, which is over 50:1 in energy terms. Only a small part of that differential results from different scales and markets. And that hydrogen is steam stripped from natural gas. Generating hydrogen by electrolysis – which will be the process when natural gas is gone – is three times as expensive.
Most experts seem to have become disenchanted with the hydrogen dream. Two recent reports almost ignore the goal of energy storage for the grid, because of the energy losses in the multiple conversions from wind or solar energy to electricity to hydrogen to electricity. They concentrate on the more immediate task of using hydrogen as a replacement for gasoline, and even there they caution against too much optimism. A National Academy of Engineering (NAE) report concludes that hydrogen can be extracted from coal and used to power vehicles, at something over twice the present price of gasoline, but concludes that the use of renewables “except possibly wind” for hydrogen production at competitive prices awaits new technologies.12 Staff writer Robert F. Service in Science recently remarked that “a hydrogen economy, if it comes at all, won’t happen soon,” and Ernest Moniz (an MIT physicist and former Undersecretary of Energy) agreed that “It’s very, very far away…Let’s just say decades, and I don’t mean one or two.”13 Other writers are if anything more skeptical. Andrew Ferguson concludes that neither wind nor biomass will be viable sources of hydrogen.
Those experts are all addressing the effort to preserve the present energy supply, at prices not too far above present levels. That probably is not an option in coming decades, and it won’t be an option in the post-fossil era. Moreover, if a way is not found to store energy inexpensively, wind and solar power will never provide a reliable energy supply. Future societies may have to adapt to accommodate periodic and unpredictable blackouts and sustained shortages in long cloudy and windless periods. It may not be our world, but the world would survive.
Scientists are working at the task of making hydrogen an economic energy carrier. They are trying everything from making electrolysis less costly by tuning the wavelength of energy, to harvesting pond scum (which releases minute quantities of atomic hydrogen) to storing the hydrogen in metal hydrides.
They are a long way from the goal.
The Long Shots
In theory, one can generate electricity from tidal energy, ocean waves and currents, geothermal vents and even the temperature gradient in the deep tropical oceans. Most of them are intermittent, but some are predictable. Geothermal energy has been a disappointment. Iceland, with its volcanic activity, gets its electricity from geothermal energy, but at the biggest site in the United States (the Geysers field in California) production has been declining 10 percent per year because of the loss of groundwater to produce steam for the boilers. An international experiment in New Mexico in the 1970s (“dry hot rocks”) undertook to pump in water to generate steam, but the experiment fizzled because the water dissipated.
Experiments with ocean wave energy have been wrecked by storms or produced only enough energy for small local projects. Opinions differ remarkably as to its potential, but it will be a costly and dispersed source of energy. In an ocean current experiment in Norway, a turbine has been placed in a strait with strong currents; it may fare better, but there are not many such locations to exploit.
The WEC lists 27 sites, worldwide, that have been studied for tidal power. It is a capital-intensive energy source. Most of the high-potential sites are in Russia, where three sites can perhaps support a total of 110,000 megawatts of generating capacity. Since the capacity factor is about 25 percent, the actual production would be more like 27,000 megawatts, but that is the equivalent of about thirty fossil fuel plants. However, that list probably exhausts the major potential sites.
The Longer Shots
Fusion power is seen by its advocates as the deus ex machina. It would run on deuterium (heavy hydrogen, which is abundant in seawater) and avoid most of the pitfalls of fission. The problem is that it is very difficult indeed to maintain a continuing process of fusion at about 100 million degrees Fahrenheit, contained only by an extraordinarily intense magnetic field. After nearly 50 years of efforts, scientists have succeeded in containing the fusion on a laboratory scale, and they have even claimed success in briefly generating nearly half as much energy as they were putting into the magnetic containment. The challenge is to show that they can generate net energy, safely and on a sustained basis, scale it up and harness it to generate electricity. The next step in the search is a proposed international experiment called the ITER. As of now, the putative sponsors have yet to resolve a bitter contest as to whether the experiment will be located in France or Japan. The path toward fusion energy has not been smooth, and there is no way of knowing whether it will ever work.
If fusion becomes workable and inexpensive, it will rewrite the whole transition scenario. With almost limitless electricity available, at no cost to the environment or the climate, we will re-enter an era of plenty, unconstrained by the limited annual input of sunlight and with none of fossil fuels’ penalties.
Let me here inject a brief aphorism: Solutions beget problems. The human race would face an entirely new set of issues. How will our economies be transformed when almost all energy is electricity, which provides neither feedstocks nor powerful, highly portable propulsion? Will the gap between the haves and have-nots intensify because poor nations and people will not be partners in this extraordinarily complex technology? Will the human race in its hubris be able to restrain its enthusiasm for cheap power? If it does not, will we drive our numbers and consumption to levels that imperil us because of the other penalties of growth? Will we imperil the other creatures on a shared planet — and eventually our own survival?
Clathrates. For completeness, let me mention the last faint hope of those who cling to the fossil era. Clathrates are globules of ice and methane widely distributed on the continental shelves of the world’s oceans, and in the tundra. It is a highly dispersed resource. To disturb the clathrates is to pose the danger of releasing the methane (which is a potent greenhouse gas) or possibly generating mudslides and tsunamis on the sloping continental shelf in the mining process, and large scale dredging would inflict damage on marine fisheries beyond anything that fishing trawlers have yet inflicted.
The scientific consensus seems to be that clathrates are a highly uncertain and potentially disruptive source of energy, but Japan in its tireless search for energy has led at least two expeditions to see if the methane can be captured.
The Energy Mix of the Future
Prof. Ted Trainer (Note 4), after an exhaustive examination of solar, wind and biomass energy and hydrogen storage, concluded that
“… implausible assumptions would have to be made before it could be concluded that present electrical and liquid fuel demand could be met from solar sources, let alone demand anticipated in view of continued economic growth. In other words renewable energy forma are unlikely to be capable of substituting for fossil fuels, and the shortfall is likely to be large, especially with respect to liquid fuels.”
“It should be emphasized again that the foregoing argument does not imply that renewable energy sources should be rejected. We should change to them as rapidly as possible, and we could live well on them but only if we accept transition to a very different society.”
That’s a pretty good statement of the issue. He might have added that decreasing population and lower demand would make the problem easier.
The broad outlines of the post-fossil energy mix may be clearer than the details.
Biomass is the only candidate that can duplicate most of fossil fuels’ roles, but its availability will be determined by future population size. A halving of world population would make it possible for biomass to provide much less than a quarter of present world energy use – but then again, energy needs would be much less than they are now. A world population of 1.6 billion – the 1900 level – would have more food per capita, and the poor majority would have access to more energy than they have now.
Wind can augment that energy, but good sites are not unlimited, and wind will not fill the fossil fuel gap. Direct solar can play a multiple role – perhaps more focused on distributed local energy and the better use of sunlight than grid energy. Direct solar energy does not have the inherent limits of the other sources, but its availability will be a function of its price and the land it requires.
Multiple local sources such as wave energy and ocean currents can further augment the supply, but sites that can pay back the energy invested in them are not common.
Most of the energy will be electricity, and that will change our economies. So will the sporadic nature of much of the energy, unless a satisfactory storage system is found, and that will put a premium on flexible, independent and small-scale energy systems.
All of the renewables, even by the most optimistic predictions, will be dramatically more expensive than today’s energy. And — unless there is fusion energy, which is still a dream — the available energy will be far less than at present. It would be an insuperable task to try to replace current sources at current scales.
The debate has been cast in the wrong terms. The problem cannot be solved if we keep asking: “What energy sources will be available to replace fossil fuels?” We should instead ask: what populations can be supported at a decent standard by the energy sources that will be available after the transition from fossil fuels? There are such sources, but they won’t be like the profligate fossil fuel era.
Talking about life styles is always tricky terrain. It can lead to maundering generalizations about the good old days, but I am serious in suggesting that we will need to adjust our goals to seek a simpler and less energy-dependent pattern of living. The models are there from the human experience, and the new technologies can improve upon them.
The Probable Impacts of the Transition
Agriculture. Modern agriculture has been called a process of using sunlight to convert hydrocarbons into food – very inefficiently. In the United States, we use about 10 calories of fossil fuels, in the form of fertilizers, pesticides and powered machinery, to produce one calorie of food. (That understates the total, since it does not include the off-farm energy.) However, we are remarkably efficient in terms of labor. Giampietro and Pimentel have calculated that it takes 166 times as much labor to raise grain in China as in the United States.14 Even with all that fossil energy, U.S. agriculture is encountering problems: declining arable land and water, faltering yields, resistant pests. American agriculture’s reliance on fossil energy during the energy transition is a catastrophic mistake. We need to find ways, as fossil fuels decline, to work the land efficiently with less energy and limited labor during a transition to lower population levels. The alternative, importing more labor from overpopulated countries, would vitiate any effort to reduce U.S. population – even as migration of people with high fertility is driving U.S. population upwards now. It is a dilemma, but we can profit from the opportunity that comes with a smaller population, to bring our national water budget in balance and to concentrate our effort on the better land.
We have separated animal husbandry from crop production. Grain production depends now on commercial fertilizers. Meanwhile, more and more chickens and hogs and cattle are raised in huge “agrifactories”, ten thousand or more at a time. Their manure is held in large holding ponds, from whence it escapes and flows toward the sea. The “dead zones” in the Gulf of Mexico, the Chesapeake Bay, and off the Massachusetts and California coasts are the product of excess chemical fertilizers and manure running into the sea, and the resultant eutrophication is destroying fisheries. Hurricane Floyd in 1999 overwhelmed the hog farm holding ponds in North Carolina and washed the manure into the Albemarle and Pamlico Sounds, with impacts on fisheries and marine life that are still being felt.
The system is fundamentally out of whack. The animals should be raised where the natural fertllizers they produce can be used on the crops. It would eliminate two sources of pollution, save energy and save the fisheries. I have run a manure spreader. Hardly glamorous work, but necessary. You don’t see them any more, but they will be back.
Diminishing fossil energy will drive up the price of chemical fertllizers. When the fossil fuels are gone, it will make no sense to harvest biomass to make commercial fertilizer when better fertilizer is already available on the farm. Some of this reversion to old practices will be driven by prices, but it would move much faster if agricultural producers were charged the pollution costs from excess commercial fertilizer and manure holding ponds.
The Chinese carry it a step farther and use human waste, nightsoil, to produce biogas and fertilizer. Chinese cities are ringed by highly productive truck farms. Fermentation can kill the bacteria and make the process safe – but China is terribly crowded. In America, dried sewage sludge is sometimes used for fertilizer, but not generally on food crops, and we do not use the biogas.
U.S. corn yields in the fifty years before commercial fertilizer were about one-quarter of present yields, wheat yields one-third. With modern agronomy, we could do better than that. We could reserve commercial fertilizer inputs for nutrients, particularly phosphorus, that tend to go down with repeated harvesting. It is hard to put a number on the appropriate U.S. population size for such a farm economy, but we supported 100-125 million people on that economy. We could probably support 125-150 million people, better, and still have enough land to produce the biomass we need. And it would be a system in balance.
Europe, Japan and the less developed countries will be making their own calculations, and they are much grimmer.
Transportation and Communication. The energy transition will profoundly change transportation and the way we live. There are now more private vehicles than adults in the United States; energy costs will drive us back to public transport. Airplanes will begin to disappear, because no fuel substitute is as cheap and energetic as petroleum. Electricity and (briefly) coal will give trains an advantage over trucks. Shipment by sea, powered by coal, nuclear – or wind – will regain the competitive advantage it had 150 years ago. Half of ocean shipping tonnage now is used to ship petroleum, and that will stop. The limits on renewable energy will force a return to more local patterns of living, working and shopping. Locally grown food will enjoy a widening price advantage over distant supplies. Telecommunications are energyefficient; they will make increasing inroads into business and personal travel.
Industry. Higher energy costs and shifting sources will drive wholesale adjustments in the economy. For example, smelted metals and building materials will get much more expensive, driving up the demand for lumber. Pharmaceuticals, plastics, and textiles, will go back to biomass and cellulose feedstocks. The cost of retooling will be considerable. Since factories’ life cycle is about 30 years, those industrialists who see the future will plan the transition early and gain an inestimable advantage over those who don’t.
Governments have a role in fostering the scientific inquiry that will help those industrialists identify the right choices.
Consumption and Equity. An effort by the rich countries to maintain present production patterns, and even more the vision of growth as a solution, will be suicidal. We will need instead a policy of accommodating the changes. The larger the population that has to be accommodated, the greater the investment needed.
The United States is regularly criticized for its high consumption levels. That particular criticism will take care of itself. High-energy prices will lead to lower material standards of living, though perhaps not of real well-being.
Will the readjustment be spread evenly? A shrinking pie intensifies the competition. Income differentials that were tolerable may become the stuff of class warfare.
Toward a More Benign System. Renewables will end the present insults to the environment, and they can be a boon if a new population transition lowers the pressures on resources. They will not affect the climate. (Even biomass absorbs and emits carbon in short term cycles.) That will not be the end of climate warming, because past and foreseeable carbon emissions will drive the warming trend for centuries. But at least it will bring the end of that warming in sight. In the title, I referred to “dawn.” Perhaps we can see the end of a potentially suicidal trajectory and the beginning of a time in which humans can live sustainably on Earth.
The Solution on the Demand Side
One of cartoonists’ stock figures is a little man with a beard, in a robe and sandals, standing on a street corner holding up a placard with the message, “Repent, for the end is near!” From time to time, I think of that little guy and ask myself, is that me? On reflection, however, I assure myself that my concerns are real. Even prophets are occasionally right. The scientists who are worried are the ones who present the weight of evidence, and their opponents generally resort to the mantras of faith, such as “they’ll find more resources.” or “The market will adjust.” (which is hardly a solution) or “Science will find a solution.” I don’t believe them.
The Two Child Family. Population growth set the scene for the overshoot scenario, and a reversal of that growth will be necessary to get us out of it. We cannot go back to the renewable energy economy of 1900 without a much smaller population. That reversal will be voluntary and manageable if we are wise, and catastrophic if we are not. It is not fated to be painful. We think of hungry peasants doing subsistence farming, but their poverty is a function of too many people on too little land. Think instead of the Amish, who work hard but live well in a largely selfcontained renewable energy system – but they have good land, and enough of it. (They also have large families, which raises the question: will fertility rise as urbanized societies de-urbanize, and children again become an economic asset rather than a burden? But I’ll save that exploration for a later time.)
In 1994, I wrote an NPG FORUM called “The Two Child Family”. The point was that stopping with two children would be a painless way to stop and reverse population growth. Because some women have no children, or one, a two-child maximum would mean a total fertility rate of about 1.5. It would not be an onerous limit. More than 70 percent of American women stop by then, even now. Let me update the graph in that article.
I had to revise the original chart. The numbers got out of date. The “unplanned” figure for 2100 (based on the Census Bureau middle projection) is now 100 million more than the 1994 projection. That is a sobering change; it shows how much faster we are growing than we thought then. We underestimated the impact of mass immigration. The need to bring population down has become more urgent and more difficult, so unlike the first graph, this one does not show population stabilization during this century. It must occur, and not too long after that, to stabilize at a better level.
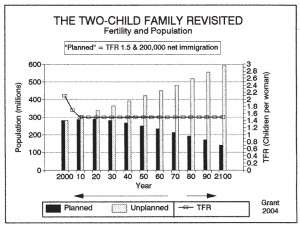
The graph is an idealization. It, too, will be overtaken if women do not decide to “stop at two.” We need a consensus that population must come down, plus the political and social will to act. I wonder whether our modern, individualistic society can act in so cohesive a fashion.
That new demographic transition is under way in Europe, Japan, South Korea, and Taiwan, where the women (if not the governments) have shown the way to smaller populations through fewer children, but they seem not to have learned yet that too few leads to extinction. Many of the so-called “less developed countries” are trying to stop population growth, but they still seek industrialization on the American pattern. That leads to a dead end, as we are just beginning to realize. They would be well advised to reconsider that objective.
The fossil fuel bubble was a durable one, and unlike soap bubbles, it will collapse slowly. That gives the world some time to make the one real accommodation that will provide a smooth transition to the leaner times ahead: a deliberate policy of negative population growth.
I do not mean to understate the difficulties, nation by nation, of learning to manage population size to maximize human welfare, or the potential for conflict as different nations move at different rates toward sustainable population levels, or the tensions created as crowded nations eye other nations’ land, water, or resources. I would not bet that the human race can manage this most difficult of transitions – this retreat from overshoot – without turmoil. But we have an opportunity to try.
NOTES:
1. See World Energy Council (WEC), 2001 Survey of World Energy Resources, Wood (including Charcoal), text and Table 9.1. It cites an International Energy Agency (IEA) estimate for all biomass which implies a figure of nine percent.
2. U.S. Bureau of the Census, Statistical Abstract of the United States, 2003, Table 899.
3. WEC, see note 1.
4. Trainer, Ted, Faculty of Arts, Univ. of N.S.W., Australia, “Renewable Energy: What Are the Limits?”, circulated by e/mail. See www.arts.unsw.edu.au/tsw/D74.RENEWABLEENERGY. html. Trainer’s study summarizes the conclusions of David Pimentel and others on net energy yields from grains and makes clear the wide variety of opinions among the experts.
5. The most cited estimate is that of Vitousek, P.M. et al, “Human Appropriation of the Products of Photosynthesis”, Bioscience, June 1986, pp.750- 760: that humans appropriate about 40% of terrestrial net photosynthetic energy.
6. Cited by Trainer (note 4).
7. An American Wind Energy Association (AWEA) estimate quoted by Andrew Ferguson,Research Director, Optimum Population Trust, UK. (www.optimumpopulation.org) suggested that the limit would be about 22 percent of present U.S. electricity consumption. (“Verdict on the Hydrogen Experiment: An Update”, draft article dated 10-20-02; personal communication.) I am indebted to Mr. Ferguson for much advice on the costs of renewable energy.
8. The ratio seems high, given other prices cited; it may include an estimate of how much it would cost to store the energy, which is still an unknown.
9. E/mail 6-29-04 from Andrew F. Ferguson (Note 7).
10. Howard C. Hayden, The Solar Fraud (Pueblo, CO: Vales Lake Publishing, 2001), p.161.
11. Hayden (Note 10), p.154.
12. National Academy of Engineering, Board on Energy & Environmental Systems, The Hydrogen Economy: Opportunities, Costs, Barriers and R&D Needs (Washington: National Academies Press, 2004), Executive Summary p.2.
13. Science, 8-13-04, p. 958. The series of articles misleadingly entitled “Toward a Hydrogen Economy”, pp.957-976, is a useful summary of the difficulties of using hydrogen for energy.
14. Mario Giampietro & David Pimentel, “The Tightening Conflict: Population, Energy Use and the Ecology of Agriculture”, NPG FORUM series, Oct. 1993.
Lindsey Grant is a retired Foreign Service Officer; he was a China specialist and served as Director of the Office of Asian Communist Affairs, National Security Council staff member, and Department of State policy Planning staff member. As Deputy Secretary of State for Environmental and Population Affairs, he was Department of State coordinator for the Global 2000 Report to the President, Chairman of the interagency committee on Int’l Environmental Committee and US member of the UN ECE Committee of Experts on the Environment. His books include: Too Many People, Juggernaut, The Horseman and the Bureaucrat, Elephants in Volkswagen, How Many Americans?